E-Sensor
Chemical Sensing for fluids in internet-of-things (IoT) is still in its infancy due to high prices for e.g. ion measurements using commercial sensor systems today. An exception are gas sensors: cost-effective CO sensors are already at a technical and price level to be used in nearly every building to prevent damages from fires in habituated houses. For liquid sensors, however, such a “killer” application for a mass market is not yet established. The gold standard, ion-selective-electrodes (ISE) are still lab-bound, constrained by the need of trained technicians and calibration, macroscopic, and thus unprofitable for the IoT and the many possible applications that open up in the next decade.
Both, the lack of cost-effective and reliable manufacturing methods and the short life times of electrochemical sensors lead to a negligence of chemical sensing of fluids - despite manifold possible applications in household, biotech, life science, agriculture, and pharmaceutical research. Additive manufacturing promises low-cost individual sensors. Recent progress in additive manufacturing opened up the possibility of single-ion sensing using a potentiometric method [1] as well as an impedimetric approach [2]. In contrast, multi-ion sensing [3], [4] is still done using conventional PVC membranes that show disadvantages in reliability, sensitivity, and repeatability of fabrication. Acrylate-based inks offer supreme homogeneity, possible fine-tuning and are predestined for low-volume additive manufacturing. The development of a ionophore-based ink suitable for ink-jet printing is a trade-off between many printing parameters, like viscosity, surface energies and adhesion properties [5]. Based on our own FEM simulation results, we managed to realize a proof of concept for measuring Na+ ions in fluids with an ink-jet- printed sensor. (currently in peer-review). In the present project, we would like extend this approach to other ionic species and take full advantage of the novel, improved fabrication method.
The ionophore-target ion systems are fully developed and commercially available as used in potentiometric sensors [6, 7]. Out of many approaches, the physical separation between target and other ionic species using ionophores succeeded. After experimentally evaluating other approaches like Cyclovoltammetry on bare Pt-electrodes, we decided to stick with this well-proven mechanism.
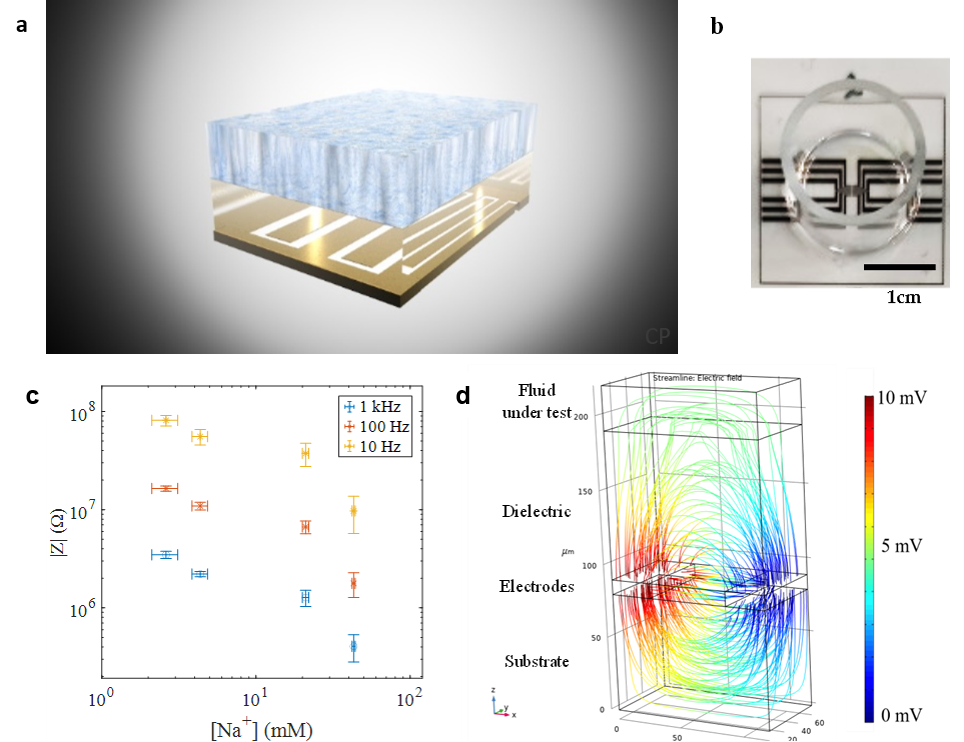
(b) Additively Manufactured Sensor Prototypes.
(c) Sensor response when sensor is wetted.
(d) FEM Simulated Field Gradients in Sensor Stack.
By improving the fabrication method, we would like to push these limitations . We choose advanced printing methods that allow much more precise, and thus repeatable resu lts than the previous gold standard of dip-coating [8]. In contrast to lithography, printing offers a wider range in the useable parameter space, for instance supporting multiple materials or creating multi-layer structures fast and more economically. Based on the literature and supported by our own experiments, ion-selective chemical sensors have to rely on a physical separation via an ionophore system [9]. Optical approaches offer detection limits down to 10-12 M, but suffer from short lifetimes mainly due to bleaching [10]. Ion selective field effect transistors (often referred to as ISFET or CHEMFETlts [8]
Compared to other ion-selective systems [13-15], our impediometric approach based on [16] and significantly enhanced with modern printing techniques offers presents an attractivea route towards more homogenous ion-selective membranes (ISM), facile fabrication, scalability, and if printable on a CMOS wWafer surface, a cheap and compatible path towards thethe integration into an easy to use integrated circuit system. The „gold“ standard comprising PVC-based, potentiometric ion-selective -electrodes usually offers low detection limits in the 10-5 M range [17]. Although some authors report lower limits (e.g. 10-7 M [18]).) Preliminary data indicates that our approach allows resolving notably lower concentrations down to 10-8 M. More importantly, we use a two-electrode setup that eliminates the need of a reference electrode by using a smart detection scheme with an analyte-insensitive reference membrane.
In general, a trade-off between (i) size, (ii) resolution/ performance, and (iii) cost has to be made. Pushing the frontier in IoT applications, a clear priority needs to be on the cost of the sensors as it is not affordable to place expensive sensors everywhere in our environment. However, electrochemical, micro Ion-Selective-Membrane- based sensors often lack the cost scalability of their fabrication process.
In a recently submitted work, we presented an additive manufacturing route for cost-effective electrochemical sensors (Fig. 1). For this tThe electrodes and the dielectric, ion-selective-membrane need to be printable. Common Ppolyvinylchlorid (PVC)- based membranes are very challenging to print in on the micrometer scale. They offer little, less biocompatibilityble and sustainabileity [12] and, even more importantly, use a solvent evaporating after deposition , and subsequently lleaving a possibly inhomogeneous layer [13]. Our polyacrylate-based ion-selective-membrane (ISM) promises a more controllable deposition method by the use of ink-jet printing. We use an impediometric measurement method with a two-electrode-setup that does not rely on a reference electrode and its drawbacks [2, 19].
[1] Christian Pfeffer, Sabine Zips, Ernst Muellner, Markus Hefele, Franz Kreupl, Bernhard Wolf, Bernhard Wolfrum, Yue Liang and Ralf Brederlow A 3D-Printable, Impedimetric Na+-Sensor for Fluids, to be published at IEEE Sensors.
[2] L. Grob, H. Yamamoto, S. Zips, P. Rinklin, A. Hirano‐Iwata, B. Wolfrum: Printed 3D Electrode Arrays with Micrometer‐Scale Lateral Resolution for Extracellular Recording of Action Potentials. Advanced Materials Technologies 5 (3) 1900517 (2020).
[3] S. Zips, L. Grob, P. Rinklin, K. Terkan, N.Y. Adly, L.J.K. Weiß, D. Mayer, B. Wolfrum: Fully Printed μ-Needle Electrode Array from Conductive Polymer Ink for Bioelectronic Applications. ACS applied materials & interfaces 11 (36), 32778-32786 (2019).
[4] P. Rinklin, T.-M. Tseng, C. Liu, M. Li, K. Terkan, L. Grob, N. Adly, S. Zips, L. Weiß, U. Schlichtmann*, B. Wolfrum: Electronic design automation for increased robustness in inkjet-printed electronics. Flexible and Printed Electronics 4 (4), (2019).
[5] N. Adly, S. Weidlich, S. Seyock, F. Brings, A. Yakushenko, A. Offenhäusser, B. Wolfrum: Printed microelectrode arrays on soft materials: from PDMS to hydrogels. npj Flexible Electronics 2 (15), 1-9 (2018).
[6] J. Schnitker, N. Adly, S. Seyock, B. Bachmann, A. Yakushenko, B. Wolfrum A. Offenhäusser*: Rapid Prototyping of Ultralow‐Cost, Inkjet‐Printed Carbon Microelectrodes for Flexible Bioelectronic Devices. Advanced Biosystems 2 (3), 1700136 (2018).
[7] B. Bachmann, N. Adly, J. Schnitker, A. Yakushenko, P. Rinklin, A. Offenhäusser, B. Wolfrum*: All-inkjet printed gold microelectrode arrays for extracellular recording of action potentials. Flexible and Printed Electronics 2, 035003 (2017).
[8] N. Adly, L. Feng, K.J. Krause, D. Mayer, A. Yakushenko, A. Offenhäusser, B. Wolfrum: Flexible Microgap Electrodes by Direct Inkjet Printing for Biosensing Application. Advanced Biosystems 1, 1600016 1-8 (2017).
[9] D. Kireev, S.Seyock, J. Lewen, V. Maybeck, B. Wolfrum, A. Offenhäusser: Graphene Multielectrode Arrays as a Versatile Tool for Extracellular Measurements. Advanced Healthcare Materials 6, 01601433 1-9 (2017).
[10] N.Y. Adly, B. Bachmann, K.J. Krause, A. Offenhäusser, B. Wolfrum, A. Yakushenko: Three-dimensional inkjet-printed redox cycling sensor. RSC Advances 7 (9), 5473-5479 (2017).
[11] B. Wolfrum, E. Kätelhön, A. Yakushenko, K.J. Krause, N. Adly, M. Hüske, and P. Rinklin: Nanoscale Electrochemical Sensor Arrays: Redox Cycling Amplification in Dual-Electrode Systems. Accounts of Chemical Research 49, 2031–2040 (2016).